Microbiological study on the management of holes in gloves for isolators
By Members of the Working Group: Barrier Technology
Marc Besson – AXYS NETWORK, Patrick Coppens – ISO’TECXEL, Edith Filaire – GROUPE ICARE, Eric Gohier – JCE BIOTECHNOLOGY, Christian Poinsot – GROUPE ICARE, Vincent Rochette – GROUPE ICARE, Antoine Toussaint – GSK VACCINES, Julien Triquet – GSK VACCINES
It is now well established that barrier systems suchbas isolators or Restricted Access Barrier System (RABS) play a fundamental role in the protection of people and products in pharmaceutical production, as well as for analytical and sterility testing (Gessler et al., 2011). Isolators
realize a separated aseptic core practically free of microorganisms, which offers maximized protection for production and testing. For the
realization of such an isolated aseptic core, considerations in microbiology play a crucial role in the validation, maintenance and control of the asepsis of an isolator classified in Grade A as per the Annex 1 requirement.
Besides, the gloves which are used to supply the crucial interface between the interior isolator environment and the worker. The integrity of the gloves is critical, so a glove breach is a breach in containment that puts products and/or workers at risk of contamination. Therefore, prevention and detection of breaches in gloves and sleeves during manufacturing operations is a key aspect of the maintenance of the barrier against contamination of the Grade A environment.
Routine glove integrity testing is mandatory as per many regulations. The US Food and Drug Administration (FDA, 2004), for example, stipulates that ‘’a fault glove or sleeve (gauntlet) assembly represents a route of contamination and a critical breach of isolator integrity. In addition, employees should visually inspect gloves for damage before each use, and physical tests should be performed routinely ». Similar standards are established in the draft of EU Good Manufacturing Practice (GMP) Annex 1 Revision V12 (2021).
The main challenge is that pharmaceutical manufacturers need to define their own standard operating procedures for glove testing according to these directives and set up an appropriate procedure for their facilities on a risk analysis basis. To our knowledge, scientific data on the pinhole
risk in gloves are scarce. The reference study is from Gessler et al., (2011), in which the relevance of pinholes to potential risks of contamination of work or product processed in isolators was quantified. At the same time, a comparison of different physical methods for glove integrity testing was realized (Flow test, pressure drop test, water breakthrough test, particle penetrating test, diffusional test, visual inspection). In this study, gloves tested were made of chlorosulfonated polyethylene (CSM), leaks being prepared using a syringe needle, having external diameters of 0.4, 0.6, and 0.8 mm, with a focus of the microorganism called Brevundimona diminuta. Even if high bioburden on the inner side of defective gloves represents a contamination risk for product as well as for the isolator interior (Grade A), the authors suggested that bioburden found on gloves in practice typically show low concentrations of < 20 CFU.cm-2.
Nevertheless, it seemed necessary not only to complement Gessler et al., (2011) study in extending the glove holes sizes to a range likely to be visible and therefore detected through visual inspection check by operators, but also to mimic the actual pharmaceutical production conditions as much as possible (such as pressure) in order to evaluate the risk of entry of microorganism (inner surface of the isolators gloves). Moreover, Maier et al., (2016) described that gloves can develop momentary pressurizations of up to 500 Pa as operators’ hands pass the more restricted wrist section, or at entry into finger pockets. Once in position, however, pressures normalize to the pressure differential as a function set for the barrier system. This momentary pressurization creates a jet stream, which comes from the surrounding environment so potentially carries both airborne particulate and microbial contamination.
Therefore, the aim of this study was to subject insulator gloves (CSM) to an aerosol containing a microorganism (Geobacillus stearothermophilus) in order to verify their tightness. Based on Gessler et al., (2011) and Maier et al., (2016), we put forward the hypothesis that below a certain size of the holes, insulator gloves can provide a seal, even if their surface is altered. Geobacillus stearothermophilus is a gram-positive thermophilic spore-formers (Burgess et al., 2017). This microorganism has been chosen, because he has the ability to grow and reproduce himself at a selective temperature not conducive to the growth of another organism, thus eliminating the possibility of false results caused by contamination by other microorganisms.
1. Materials and Methods
Pinholes in Gloves
Fifteen CSM gloves (size: 8; thickness: 6 mm) were tested. Calibrated holes of different diameters (1, 2 and 3 mm) using a cookie cutter were
realized, so that the holes are always positioned in the same place, at 1cm from the fingertip. These diameters were chosen because these are size
visually inspectable (that an operator with eye can see).
Evaluation of the pression induced by the introduction of the hand in the glove
After placing the glove on a wrist ring connected to a cuff and cleaning it with isopropyl alcohol, the hand was introduced into the glove and the
pressure was taken continuously at the tip of the finger (middle finger) and in the palm of the hand thanks to a Delta ohm (Figure 1).
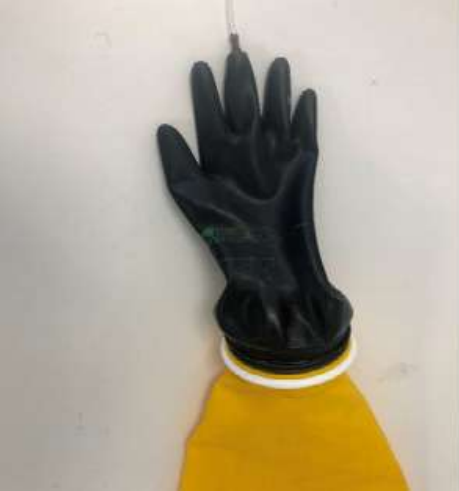
Preparation of the suspension of Geobacillus stearothermophilus spores ATCC 7953
A suspension of Geobacillus stearothermophilus ATCC 7953 adjusted to approximately 107 CFU.ml-1 was used.
Nebulization of the suspension
Two isolators meeting the qualification standards of the pharmaceutical industry were used (Figure 2). The glove to be tested was placed on the wrist ring on the left door of the transfer lock between the two isolators, glove hole being directed towards the back wall. After a glove predecontamination with isopropyl alcohol, a Vapour Phase Hydrogen Peroxide (VPHP) decontamination of the two isolators was carried out for 15 minutes. Then, VPHP decontamination was stopped and a ventilation time was applied to decrease the HP air rate to 0 PPM. The nebulizer tank was filled by 7 ml of the Geobacillus stearothermophilus ATCC 7953 and the nebulization was started in the right-hand side isolator. Once the nebulizer tank was empty, the pressure was increased to 500 Pa in the right chamber in order to mimic the overpressure induced by the introduction of the hand into the glove, and to facilitate the passage of the spores through the glove.

Figure 2 : Two isolators connected by a transfer lock and previously decontaminated by H2 O2 are used. The glove to be tested was placed on
the wrist ring on the left door of the transfer lock between the two isolators, glove hole being directed towards the back wall. The Geobacillus stearothermophilus ATCC 7953 nebulization was started in the right isolator. Once the nebulizer tank was empty, the pressure was increased to 500 Pa in the right chamber in order to mimic the overpressure induced by the introduction of the hand into the glove. Swab around the hole on the index finger of the glove tested were realized and spread on Trypticase Soy agar (TSA). Surface sampling using count-tact boxes on the walls of the left isolator were also done.
Microbiological test
Swab around the hole on the index finger of the glove tested was realized and spread on Trypticase Soy agar (TSA). Surface sampling using counttact plates on the walls of the left-hand side isolator was also done. After 2 days incubation at 58 ° C ± 2 °C, the presence or absence of colonies was evaluated.
2. Results




Firstly, the inhibitor effect of HP on packed culture media was evaluated. For that, culture media, which were packaged in releasable plastic bags, were exposed to VPHP for 40 minutes. Table 1 presents the comparison between media exposed and not exposed to VPHP.
The recovery of colonies was similar whatever the treatment, reflecting no inhibitor effect of HP on packed culture media.
Focusing on the pressure induced by the introduction of the hand into the glove, we noted that the differential pressure is on average 435 Pa. This was in agreement with those found by Mayer et al., (2016).
Results of the microbiological test are shown in Table 2, 3, and 4.
Three major effects can be seen:
- Bioburden found on the surface sampling on the isolator was proportional to the size of the hole.
- Bioburden found on the gloves with a hole of 1mm is low, the average value being 11 CFU. cm-2, initial bioburden108
- Focusing on the 1 mm diameter hole and after checking their size using a binocular magnifier glass, we could see that the holes ranged from 0.53 to 1 mm (Table 3). Moreover, up to 0.65 mm, the microbial load is very low.
Discussion
The nature of the gloves, due to their thickness and their flexibility, induces a risk of tearing or piercing. It is therefore considered to be one of the main sources of contamination. Minimizing breaches in the gloves is therefore a significant safety concern in daily operations across life sciences applications. Even if the pharmaceutical industry agrees with the requirement of testing gloves, there is still a debate concerning the pinhole size in gloves, that can induce a breach in isolator integrity and passage of microorganisms. The aim of our study was to use bioburden conditions close to industrial reality in class C to assess the transfer through very visible hole sizes. The ultimate goal was to give arguments to manufacturers to develop their own Standard Operation Procedures and decisions based on Quality Risk Management principles. Under the chosen experimental conditions, our results showed that under a size of hole about 0.6 mm, defective gloves have a low probability to represent a microbiological risk.
Beyond this size, microbial contamination seems to increase.
These results are in agreement with those of Gessler et al., (2011). However, they give additional points of view insofar as the protocol tries to reproduce the real conditions of the industry. It is also important to note that the bioburden nebulization used in our study before the pressure pulse at 500 Pa, is about 108 spores. According to production standards in the pharmaceutical industry, such a bioburden is, to our knowledge, never present in the production environment. Thus, probability that microorganism passage will pass is therefore almost null. Nevertheless, further investigations are needed, including hole size range evaluation on other glove materials, and other bacterial strains evaluation, combined with visual inspection.
References
- Burgess SA, Flint SH, Lindsay D, Cox MP, Biggs PJ (2017) Insights into the Geobacillus stearothermophilus species based on phylogenomic principles. MC Microbiol. 26; 17 (1): 140.
- Food and Drug Administration (2004) Guidance for Industry: Sterile Drug Products Produced by Aseptic Processing – Current Good Manufacturing Practice.
- Gessler A, Stärk A, Sigwarth V, Moirandet C (2011) How risky are pinholes in gloves? A rational appeal for the integrity of gloves for isolators. PDA J. Pharm. Sci. Technol. 65 (3): 227-41.
- Maier C, Drinkwater JL (2016). Managing contamination risks in glove holes in barrier separation technology. European Pharmaceutical Review.

For more informations, click here